Exotic Modulation – Beyond 8-VSB
There are currently some new, exotic modulation methods under development. Although these methods are not likely to be used for traditional broadcasting, the technology behind them is interesting and will affect broadcasting in one way or another in the years to come. First, however, some comments on the status of the U.S. DTV modulation standard.
After considering the results of DTV field tests in two cities, the Association for Maximum Service Television (MSTV) and the National Association of Broadcasters (NAB) concluded, "there is insufficient evidence to add COFDM and we therefore reaffirm our endorsement of the VSB standard." Consumer electronics manufacturers and the FCC welcomed the endorsement. What was not widely reported was another statement made in the MSTV and NAB resolution: "We also conclude that there is an urgent need for swift and dramatic improvement in the performance of the present U.S. digital television system."
Ten days after the resolution was released, the Advanced Television Systems Committee (ATSC) Specialist Group on RF Transmission issued a Request for Proposals (RFP) for enhancements to the ATSC transmission specifications.
It appears that neither COFDM nor 8-VSB as tested offered satisfactory reception with indoor antennas. Note that the majority of COFDM DTV installations in England rely on outdoor antennas. Perhaps we’re asking too much of a DTV standard to provide reliable indoor reception, in what has been characterized as a weak-signal, multipath-degraded environment, at a payload data rate of 19.3 Mbps.
If reliable reception on indoor antennas and portable sets can’t be achieved with 8-VSB at 19.3 Mbps, some implementation of hierarchical modulation (or scalability) is needed in the ATSC standard. In comments to the FCC and Congress last year, some manufacturers indicated this was under development. It will be interesting to see what the response is to the ATSC RFP and what, if any, progress on a scalable ATSC system is announced at NAB2001.
Another alternative is diversity reception. The use of diversity or smart antennas for DTV has been suggested. Perhaps the algorithms used for the Bell Labs BLAST system, I’ll describe later, could be used to enhance 8-VSB reception in a multipath environment.
MODULATING TIME – ULTRAWIDEBAND
If you follow the FCC’s actions, you may have noticed there has been increasing activity related to ultrawideband technologies. The implementation I’ll discuss is the PulsON technology from Time Domain Corporation (www.timedomain.com). While the system has yet to be demonstrated at data rates that would work for HDTV, and the chances of it replacing our established analog and digital modulation methods for broadcast TV are slim, I found the PulsON technology intriguing. As this is an ultrawideband system, there are some concerns it could interfere with TV broadcast reception, microwaves and GPS receivers.
Time Domain’s PulsON is based on a time-modulation ultrawideband (TM-UWB) technology where trains of short pulses are transmitted at very precise time intervals (10-picosecond accuracy). The short pulse is a Gaussian monocycle – imagine one cycle of an RF signal – with a width between 0.20 and 1.50 nanoseconds. The shape of the pulse and the short duration make it inherently wideband. A monocycle with a center frequency of 2 GHz will have a frequency distribution with a 3 dB bandwidth of more than 2 GHz.
The monocycles themselves do not carry the information. UWB is often confused with spread-spectrum systems, where information is distributed over a number of frequencies, usually with a pseudorandom spreading sequence. In UWB systems, information is not coded in the amplitude or frequency of the monocycle(s). Instead, the information to be transmitted is encoded in the positions of the pulses.
The receiver consists of a highly accurate clock oscillator and a correlator that multiplies the incoming signal with a "template" waveform to extract time differences between the incoming signal and the template. Because there is no "carrier," there is no need for a local oscillator, intermediate frequency (IF) amplifier or tuned circuits. Maintaining the 10-picosecond clock accuracy, however, is not trivial. The transmitter could be as simple as a digital processor/modulator driving a single high-speed switching transistor or FET with an output filter to shape the monocycle’s frequency response.
SIMPLEST FORM
In its simplest form, a 10-million-pulse-per-second (10 Mpps) system would transmit a monocycle every 100 nanoseconds. Modulation could be applied by transmitting a pulse 100 picoseconds early to represent one binary state and 100 picoseconds late to represent the other. However, because the position shift is small, such a transmission would have energy peaks at certain repeating frequencies, depending on the modulation rate.
To better disperse the energy and reduce interference to other services, Time Domain’s system uses a pseudo-random code sequence to shift the pulses by several nanoseconds. The result is the transmission has a more noiselike spectrum. By using different pseudo-random code sequences, a large number of transmissions can share the same spectrum.
Keep in mind that while this sounds like spread-spectrum, conventional spread-spectrum systems either hop between frequencies or have the data spread out from a single frequency carrier using a pseudo-random code sequence. The PulsON system hops between time slots, and there is no "carrier" – only the monocycles.
PROTOTYPE SYSTEM
How well does the system work? Time Domain Corp. says it has built a prototype system that operates over spectrum centered around 1.3 GHz at 250 microwatts output. It has been successfully tested over distances beyond 10 miles at data rates of 39 kbps or 159 kbps.
Multipath is not a problem. Because the position of the pulses is so accurately determined, a rake receiver (visualize it as a receiver that will intercept pulses only at a fixed position on the "rake") can easily separate the main pulse stream (if any) and the various reflected pulse streams. If more than one correlator is used, multiple pulse streams from the transmitter, arriving over multiple paths, can be detected, aligned and combined, increasing the signal-to-noise ratio compared with that of a single path. In this case, multipath improves reception.
Time Domain said it has implemented a timer and a correlator chip using Silicon Germanium technology and has a CMOS logic chip under design, so UWB products could start appearing soon. Interference concerns, however, will limit widespread use of the technology until it can be proved that other services will not be degraded.
How much interference would a UWB system create? At the power levels being considered and with sufficient coding to avoid creating energy peaks at specific frequencies, there is very little chance it would interfere with terrestrial TV reception – either analog or digital.
As a noiselike signal, it would of course slightly raise the threshold for DTV reception. The major interference concern has been with GPS receivers, which work at very low carrier-to-noise ratios. The FCC has granted Time Domain Corp., Zircon and U.S. Radar Inc. waivers to allow limited marketing of UWB devices, with severe restrictions on their use.
MODULATING SPACE – BLAST
Bell Labs has developed a novel way to increase spectrum efficiency (more bits per Hertz) without affecting reliability. Unlike UWB, parts of the Bell Labs technology may be used to improve DTV reception in the presence of multipath. If you closely followed the 8-VSB versus COFDM debate, you probably heard the argument that in urban areas the Rayleigh propagation model applies, while 8-VSB was designed to work in a Ricean environment. A Rayleigh environment is one where there is no dominant signal path. A Ricean environment is one characterized by a one dominant path, although other weaker paths may exist. Bell Labs Layered Space Time technology or "BLAST" (www.bell-labs.com/project/blast/) is designed to work in a Rayleigh environment.
BLAST uses multiple transmitting antennas and multiple receiving antennas. While each antenna transmits on the same frequency, the datastream is split into multiple substreams that are transmitted in parallel, one substream per antenna. The effective data transmission rate is increased by an amount roughly proportional to the number of antennas used.
The transmission side of the BLAST system is easy to understand. Of course, for it to be useful there has to be a way to receive the individual datastreams and recombine them. Multiple antennas are required at the receiver as well as the transmitter. However, where each antenna on the transmission side is carrying an individual data substream, on the receive side the antennas will intercept all the data substreams.
How do you separate them? Bell Labs’ BLAST High-Level Overview explains that if there is sufficient scattering due to multipath, because the substreams are transmitted from antennas at slightly different locations in space, the substreams will be scattered differently. The Bell Labs Overview said, "Using sophisticated signal processing, these slight differences in scattering allow the substreams to be identified and recovered. In effect, the unavoidable multipath is exploited to provide a very useful spatial parallelism that is used to greatly improve data transmission rates." It also noted that when using the BLAST technique, "the more multipath, the better."
The BLAST signal processing algorithms simultaneously work on the signals from the receive antennas. First, the strongest substream is extracted. Once the strongest substream is identified, the algorithm can remove it from the collection of signals, allowing the weaker substreams to be identified and extracted. Laboratory prototypes have been built with spectral efficiencies of 20 to 40 bits per second per Hertz of bandwidth.
A SIMILAR APPROACH
WJ Communications (www.wjcommunications.com) appears to be taking a similar approach with a system it has developed. Very little technical information was available about the development on the WJ Communications Web site, but a line in the press release announcing the technology’s ability to exceed the data rate limit imposed by Shannon’s law said, "The creation of extra-dimensionality between transmitter and receiver is achieved by properly sampling the wavefield space created by the multipath propagation environment."
WJ Communications said it has demonstrated transmission of data in the range of 60-155 Mbps in a bandwidth of 2-4 MHz. This places its spectral efficiency in the same range as the BLAST technology.
What do these new modulation techniques mean to broadcasters?
First, we may have to find alternatives to our conventional means of sending video and audio using spectrum-wasting FM modulation as other services adopt new, spectrum-efficient technologies. Other services have an eye on our broadcast auxiliary spectrum. If we appear to be squandering spectrum, they will push for reductions in the bands.
This has already happened in the 2 GHz band, and the 7 GHz band is likely to be the next target. At this time, UWB is primarily useful for short-range work at relatively low data rates, so it isn’t a real alternative yet for TV transmission. Techniques like BLAST, however, could be very useful in maximizing use of the shrinking broadcast auxiliary spectrum.
Second, the algorithms used at Bell Labs for BLAST and at WJ Communications may modified to provide much more robust reception of 8-VSB signals in a multipath environment. Multiple receive antennas would be required, but at UHF they wouldn’t take up much space. Use of a vertically polarized component (circular or elliptical polarization) on the transmit side should also help. Bob Plonka from Harris discussed this in a paper at NAB a few years ago.
As always, your comments are welcome. Write me at dlung@transmitter.com and visit my Web site at www.transmitter.com or www.xmtr.com.
The professional video industry's #1 source for news, trends and product and tech information. Sign up below.
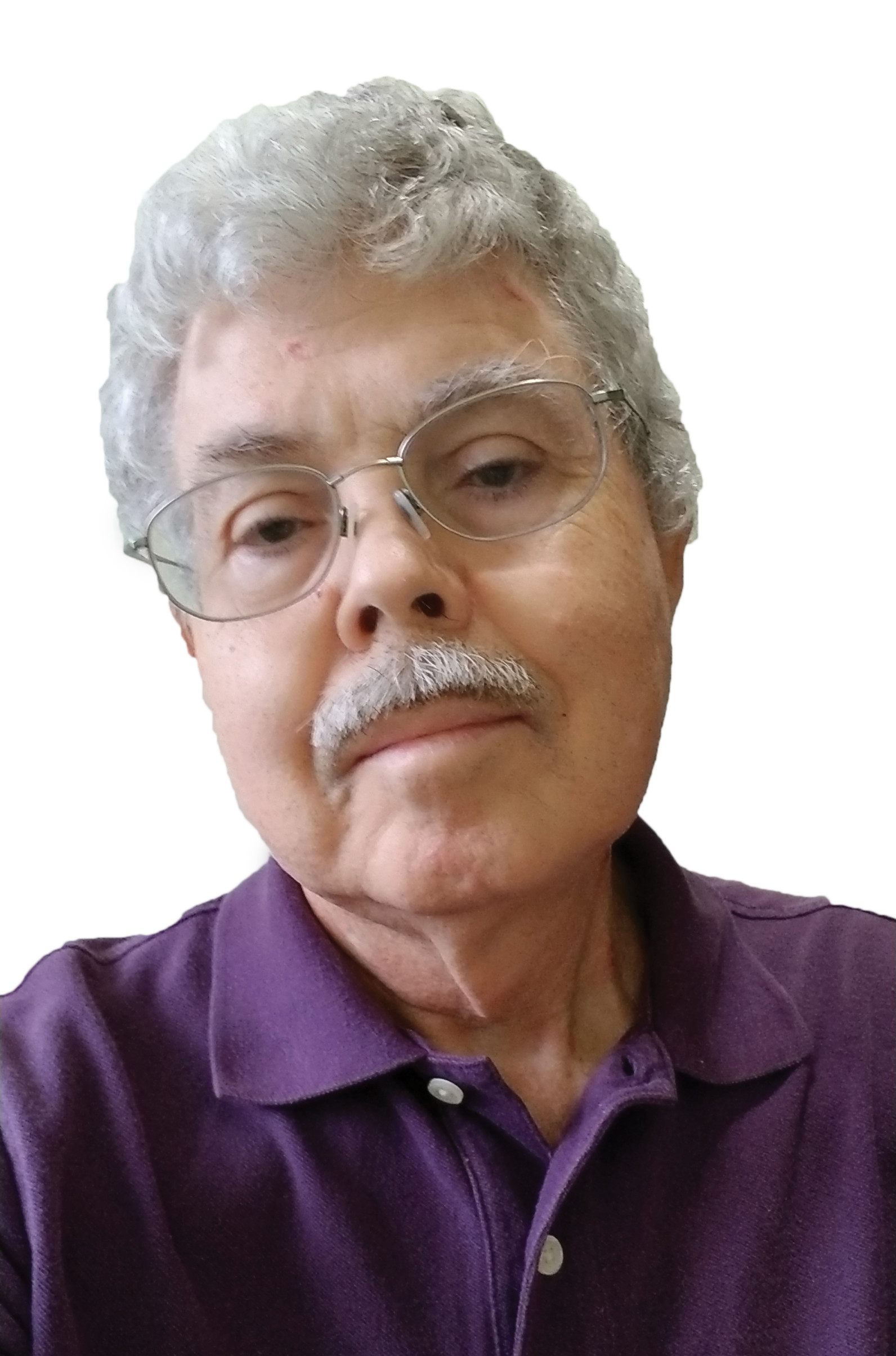
Doug Lung is one of America's foremost authorities on broadcast RF technology. As vice president of Broadcast Technology for NBCUniversal Local, H. Douglas Lung leads NBC and Telemundo-owned stations’ RF and transmission affairs, including microwave, radars, satellite uplinks, and FCC technical filings. Beginning his career in 1976 at KSCI in Los Angeles, Lung has nearly 50 years of experience in broadcast television engineering. Beginning in 1985, he led the engineering department for what was to become the Telemundo network and station group, assisting in the design, construction and installation of the company’s broadcast and cable facilities. Other projects include work on the launch of Hawaii’s first UHF TV station, the rollout and testing of the ATSC mobile-handheld standard, and software development related to the incentive auction TV spectrum repack. A longtime columnist for TV Technology, Doug is also a regular contributor to IEEE Broadcast Technology. He is the recipient of the 2023 NAB Television Engineering Award. He also received a Tech Leadership Award from TV Tech publisher Future plc in 2021 and is a member of the IEEE Broadcast Technology Society and the Society of Broadcast Engineers.