Comparing 8-VSB and COFDM for DTV Broadcasting
After reading last month's column you may have concluded that the DVB-T COFDM transmission system had enough advantages over the ATSC 8-VSB system to justify using it to replace or supplement 8-VSB. This, of course, would depend on where indoor reception ranked on your station's list of priorities. There are trade-offs, not all of them technical, in changing DTV transmission systems.
Meanwhile, new 8-VSB demodulator chips announced late in August offer the promise of significantly reducing some of the trade-offs broadcasters have had to accept with the 8-VSB standard. As demonstrated in the tests by Sinclair in Baltimore, multipath -particularly dynamic (changing) multipath - has turned out to be a significant problem for 8-VSB reception with today's DTV tuners.
This month I'll look at the new chips, outline how you can measure simple multipath and wrap up with what I hope you will consider a brief, but unbiased, comparison of the trade-offs between 8-VSB and COFDM.
NEW CHIPS TACKLE MULTIPATH
The Sinclair 8-VSB and COFDM demonstrations in Baltimore this summer caused many broadcasters to wonder if indoor reception of 8-VSB was possible. The performance of the ATSC receivers tested was not up to that of the DVB-T receivers used in the test. While there were complaints that the DVB-T receivers used were designed for professional use rather than consumer DTV reception, the poor performance of the 8-VSB receivers under the test conditions was undeniable.
Useful LinksMotorola Semiconductor Products
NxtWave
Dielectric Communications
Hammett & Edison Filing on Biennial Review of Proceeding 87-268 on DTV
Consumer Electronics Manufacturers Assoc.
ATSC
Doug Lung's RF Technology PageDTV reception fails when a receiver is unable to recover the datastream being transmitted. This can happen for several reasons, but for indoor reception in a strong signal area, it is most likely to happen when the DTV demodulator is unable to lock to the incoming datastream. While this may sound obvious, it is important to look at why the receiver can't recover the datastream clock and synchronize to it.
This summer it was discovered that the ATSC encoder itself could cause reception problem! Specifically, many consumer DTV sets were unable to lock to the signal when the SMPTE-310 ATSC datastream applied to the transmitter modulator had excessive clock jitter.
The jitter may be caused by an unlocked nonprecision oscillator in the ATSC encoder or phase variations in the STL path. This problem could not be seen on the Tektronix RFA300 or Hewlett-Packard HP89441 signal analyzers.
That problem is easy to fix. The other problem, multipath, isn't. Anyone who has looked at the waveform display of the 2T pulse or 1T bar in the FCC composite VITS from an NTSC signal knows what multipath can do to pulses. On an 8-VSB signal, multipath will distort and smear the data transitions. The demodulator won't find the transitions where it expects them and won't lock up.
You may remember from my 8-VSB basics column last year that the ATSC signal is designed to make it easier for demodulators to lock to the signal. First, carrier recovery is done using the pilot signal in the data. Second, the clock signal is extracted from a data sync segment transmitted every 77.3 microseconds. Finally, a framing code segment is transmitted every 24.2 milliseconds.
DATA DEMODULATION
Once the demodulator has acquired the framing code segment, it can use the pseudo-random sequence in it to control an adaptive equalizer to cancel out the effect of the multipath, allowing accurate data demodulation.
If the multipath delay is changing, the demodulator will have difficulty keeping the adaptive equalizer optimized (if it is able to recover the signal at all) if it must depend on the framing code. There is a solution to this, but it apparently has not been well-implemented, if included at all, in the current generation 8-VSB DTV tuners. That solution is what is called "blind adaptive equalization."
The MCT2100 and NXT2000 ATSC 8-VSB receiver chips from Motorola and NxtWave, respectively, include technology developed by Sarnoff Laboratories for blind adaptive equalization. The advantage of blind adaptive equalization is that it adjusts the adaptive equalizer using only the received data sequence. Detection and decoding of the framing code segment is not required.
By this time, all readers should be familiar with what an 8-VSB signal looks like ÷ random noise with the same average level over a 5.3 MHz bandwidth. It would appear that it should be easy for a chip to "flatten" the response.
Appearances, as usual, are deceiving. First, one multipath signal will affect more than one frequency in the channel. Second, the phase of the multipath signal has to be considered. Third, while the 8-VSB signal looks stable on a spectrum analyzer, this is because it is being averaged, either digitally or by phosphor persistence. An adaptive equalizer can't ignore the changes the averaging hides.
BLIND EQUALIZATION
Still not convinced of the difficulty in developing a reliable blind equalization system? Talk to a transmitter engineer, who was around in the mid- to late-1970s, about his or her experience with the Datatek equalizer. This was a two-rack-unit device with a front panel completely covered with knobs.
As I recall, with the exception of one or two knobs, they all controlled the equalization at a specific time ranging from about 100 nanoseconds to more than a microsecond, both before and after the main signal. With care, you could hide all sorts of sins in the transmitter and antenna at the price of some extra noise.
However, if you tried to adjust the knobs to get a flat frequency response, the pulse response would often look horrible. After a bit of experimentation, you would find that if you adjusted the unit so the pulse response looked good, the frequency response would follow.
Equalization is where the MCT2100 and NXT2000 chips offer a major breakthrough. These chips are designed to perform blind adaptive equalization under dynamic multipath conditions. Both are capable of equalizing multipath over a range from -4 to +44 microseconds.
Matt Miller, president and CEO of NxtWave boldly stated: "With the NXT2000 weâve cracked the code for indoor and mobile reception, shown that VSB is a robust and commercially viable standard, and paved the way for mass market deployment of error-free digital TV."
Mike Neshat, NxtWave vice president of marketing and sales, explained, "We designed a completely new equalizer architecture that not only overcomes the severe impairments in broadcast channels, but also provides shorter acquisition times and reduces silicon cost." Signal acquisition time was quoted at 50 milliseconds or less. If the MPEG decoder cooperates, this should allow channel surfing.
The data sheets available on the NxtWave Web site did not include any details on dynamic multipath performance. However, that is a given if the chip is going to work in indoor and mobile reception environments.
IMPRESSIVE TEST RESULTS
Frank Elroy, with Motorola's Digital TV Operations, took time to explain Motorola's MCT2100 chip in more detail. He said the MCT2100 had been tested with static echoes up to 0.1 dB below the desired signal. Even with the echoes, several decibels of signal-to-noise ratio (SNR) above the threshold of visibility remained.
This is impressive, because an echo of this amplitude will create spectral notches of more than 20 dB across the channel. The phase or delay (within the stated range) is not important, unless one of the spectral notches ends up precisely on the pilot carrier. However, as Elroy pointed out, this would simply require a one-time realignment of the antenna to move the notch off the pilot.
You may recall from last month's discussion that the DVB-T COFDM transmission system can recover data even when several carriers in the channel are completely destroyed, as would happen with a zero dB echo. In the real world, it is unlikely the amplitude of an echo would fall within 0.1 dB of the desired signal. Therefore, with regards to static multipath, if the Motorola MCT2100 performs as well in the real world as in the tests, COFDM would appear to offer little advantage over 8-VSB.
Dynamic multipath has been considered the major problem with indoor reception of 8-VSB DTV. In testing the MCT2100, Motorola used an ensemble of dynamic echoes designed to emulate the Sinclair "Lombard Street" measurements. In this emulation phase changes of 10 Hz were tested.
The MCT2100 handled it without difficulty. Motorola's Elroy explained, "After the success of that test, the largest echo (measured at -8 dB by Sinclair/Oak Technology) was increased to -6 dB, still at 10 Hz phase variation. SNR margin above TOV was maintained for this 'Lombard Street Plus' ensemble. In this dynamic multipath test, 15 dB notches ripple through the spectrum, so we also see the pilot amplitude changing by 15 dB. This is not an issue for pilot tracking, since the pilot is visible with some regularity and no antenna adjustment is required."
THE HARBOR ADJUSTMENT
Motorola also tested an emulation of the "Harbor Apartment" echo ensemble, where the largest echo was -4 dB with several smaller echoes out to 23 microseconds. A margin of several dB over threshold of visibility was measured, even when a 10 Hz phase variation was applied.
Frank Elroy was careful to point out that echo performance is more complex than this. "The performance is a complex function of the entire echo ensemble and we are still characterizing this performance space of echo amplitudes and dynamic phase changes."
MEASURING MULTIPATH
If you have access to an HP89441V signal analyzer, it is possible to download the equalizer tap data from the instrument to determine multipath. Some of the professional ATSC 8-VSB demodulators also offer this function.
Here is a simple method for calculating multipath using an instrument most TV stations have ÷ a spectrum analyzer. If only one echo is present, the measurement is straightforward. If multiple echoes are present, it should still be possible to use this method, if the echoes are not spaced too close. An example should make it easier to understand the effect of multipath on DTV reception.
Echoes, or "ghosts," on analog signals are easy to measure, particularly the longer ghosts. The ghost will appear as a second sync bar or repeat of a picture feature, such as the leading or trailing edge of the 1T bar in the FCC composite test signal, a vertical line in the picture or text character in the picture.
Measure the distance between the ghost and the main signal and you will know the multipath. This can be done directly on a waveform monitor or even by measuring the distance on a TV screen and working out the ratio between it and the approximately 50 microseconds of visible picture.
Digital signals don't provide a convenient reference point. However, because the echo signal is a copy of the desired signal, simply delayed, when it is added to the desired signal it will add or cancel at specific frequencies, depending on the delay and phase.
FREQUENCY RESPONSE
If you've looked at a plot of the return loss of an antenna, you've probably noticed a ripple in the frequency response similar to that shown in Fig. 1 (although at a much lower amplitude!). The frequency spacing between the maximum (or minimum) of each ripple is inversely proportional to the delay of the reflection. This can be used to determine where the mismatch is located on a transmission line (after considering velocity factor) or how long the multipath is on an off-air signal. The formula is simple:
Delay = 1/Ripple_Frequency_Difference
For the range we are working with, if we measure the delay in microseconds, the frequency difference between ripple maximum (or minimum) will be the reciprocal of that, or megahertz.
With this information, we can calculate the multipath present in the nonartist conception of an off-air 8-VSB DTV signal with one echo (shown in Fig. 1). Observe that the spacing between each cycle of the ripple is 1.5 MHz. The echo delay in this case will be the reciprocal of that, or 0.667 microsecond.
Note that as the echo length increases, the frequency spacing will decrease. If the echo delay were 1.0 microsecond, the frequency spacing between ripple cycles would be 1.0 MHz.
This plot can also be used to calculate the amplitude of the echo. While Fig. 1 gives the impression that the ripple is symmetrical about the average power level, that isn't really the case. For example, if we had that elusive zero dB echo, the minimum would be nothing (no signal) and the maximum would be three dB more than the "main" signal.
I won't take time to discuss the method for calculating echo level in detail, but this somewhat roundabout example may make it easier to understand, particularly if you are familiar with transmission line measurements. Convert the maximum and minimum ratio in dB to a voltage ratio before doing the calculations. We'll call this "SWR" for the example here. The equation then becomes:
Echo = 20 Log SWR -1/SWR + 1
In our example, the maximum-to-minimum power ratio is 20 dB, or 100 to 1. Expressed in terms of voltage, the ratio is 10 to 1, so SWR = 10. Substituting that number in the equation above, we calculate the echo level in Fig. 1 as -0.92 dB. If the formula looks familiar, it is because if SWR is set to the standing wave ratio on a transmission line, the formula returns the reflection coefficient.
PRACTICAL CONSIDERATIONS
I encourage readers to do their own research comparing 8-VSB and COFDM. The references in last month's RF column are a good start. Consider the arguments you hear from both sides with the same skepticism as you would a sales pitch on a new product at NAB. For example, interference and coverage issues are near the top of the debate, but a recent ATTC study showed the original DTV interference criteria do not apply at higher signal levels.
Dielectric's Oded Bendov questioned the validity of Longley-Rice for DTV coverage studies, especially in shadowed areas in a paper presented at NAB â99. In a recent filing with the FCC, Hammett and Edison pointed out errors in the FCC's DTV Table of Allotments due to the method for dealing with Longley-Rice error-code-3 cells as well as several other issues. If you agree with these conclusions, many of the coverage and interference concerns expressed about COFDM seem less significant.
On the other hand, manufacturers of receivers and transmitting equipment alike are lining up to say it's too late in the process to switch standards. Is it worth considering this disruption when devices like the chips mentioned earlier promise indoor 8-VSB reception close enough to COFDM to make little practical difference?
Other DTV field tests with indoor antennas in Raleigh and Chicago fared much better than those in Baltimore. The DTV power penalty due to the higher peak-to-average ratio required for COFDM is real. It isn't an issue in a 50 kW ERP test, but it will be an issue for stations struggling to hit 1,000 kW average DTV ERP.
If these stations must reduce power, how will COFDM coverage compare with the 8-VSB coverage promised by the next generation of receiver chips?
Much of the argument for 8-VSB, outside the disruption caused by changing transmission standards in the middle of the DTV transition, depends on these and other new receiver chips meeting their promised performance criteria. Having the technology isn't enough. It has to get into the hands of consumers, at a reasonable price.
Motorola said it expects the MCT2100 will start appearing in set-top boxes and PC card tuners in the first quarter of next year. If the company stays on schedule, we should know by then whether the promise has been fulfilled.
Drop Doug Lung a note atdlung@transmitter.com
Get the TV Tech Newsletter
The professional video industry's #1 source for news, trends and product and tech information. Sign up below.
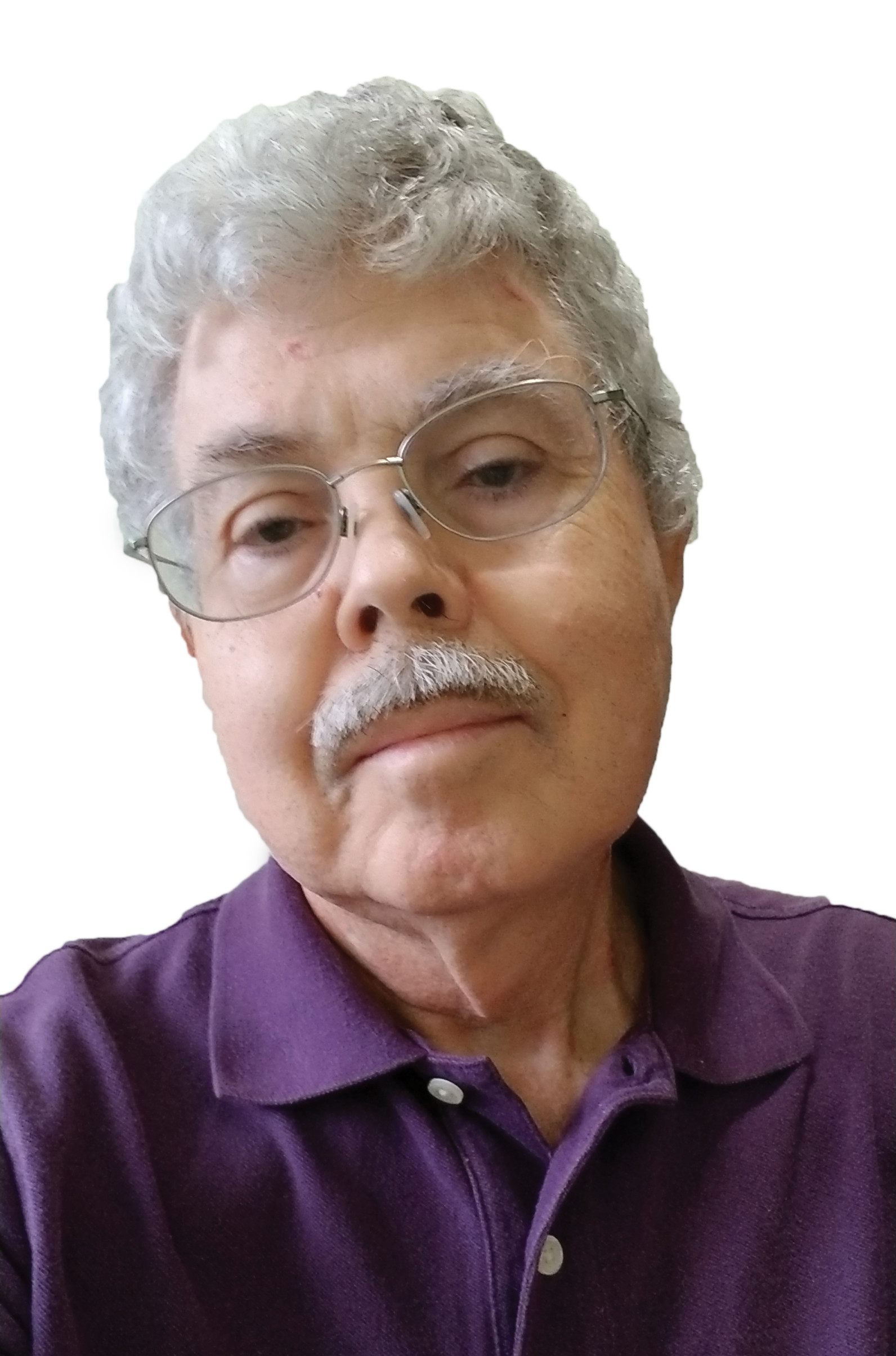
Doug Lung is one of America's foremost authorities on broadcast RF technology. As vice president of Broadcast Technology for NBCUniversal Local, H. Douglas Lung leads NBC and Telemundo-owned stations’ RF and transmission affairs, including microwave, radars, satellite uplinks, and FCC technical filings. Beginning his career in 1976 at KSCI in Los Angeles, Lung has nearly 50 years of experience in broadcast television engineering. Beginning in 1985, he led the engineering department for what was to become the Telemundo network and station group, assisting in the design, construction and installation of the company’s broadcast and cable facilities. Other projects include work on the launch of Hawaii’s first UHF TV station, the rollout and testing of the ATSC mobile-handheld standard, and software development related to the incentive auction TV spectrum repack. A longtime columnist for TV Technology, Doug is also a regular contributor to IEEE Broadcast Technology. He is the recipient of the 2023 NAB Television Engineering Award. He also received a Tech Leadership Award from TV Tech publisher Future plc in 2021 and is a member of the IEEE Broadcast Technology Society and the Society of Broadcast Engineers.