Single Frequency Networks for DTV Part I
In my December column I predicted that the future of free terrestrial over-the-air DTV would depend on reliable set-top antenna reception. Single-Frequency Networks were discussed at the 2002 IEEE Broadcast Technical Symposium as a way to improve DTV reception. Over the next two months, I'll focus on this technology and discuss some of the challenges in implementing it.
The concept of a single-frequency network with multiple transmitters is at odds with the traditional broadcasting practice of using one high power facility to cover a wide area, although it has been adopted by other services. Since broadcasting began more than 75 years ago, with only a few exceptions stations have relied on one big transmitter to reach their primary audience. With the introduction of DTV, more broadcasters are starting to ask if multiple transmitters might make more sense.
WHAT IS A SINGLE-FREQUENCY NETWORK?
The simple definition of a Single-Frequency Network (SFN) is a collection of transmitters operating on the same frequency carrying the same information to receivers in a given area. This could be a collection of on-channel boosters. These are relatively simple, low-power devices that receive a signal off-air, perhaps process it to correct transmission errors, and retransmit it on the same channel. There has to be sufficient isolation between the input of the booster and the output to prevent it from going into oscillation. While steps can be taken to locate the booster where it won't interfere with the signal from the main transmitter, there usually is no way to change the timing of the booster to prevent it or the main transmitter appearing as an echo outside the correction range of a DTV receiver.
A more complex distributed transmission system, usually with higher power transmitters, is fed from a common location. This network can be improved by adjusting the timing of the signal from each transmitter to minimize multipath from other transmitters in the network.
SOLVING RECEPTION PROBLEMS WITHMULTIPLE TRANSMITTERS
Why would anyone want to use multiple transmitters instead of one big transmitter?
Consider an indoor antenna in a house. If the big transmitter is blocked from the antenna by the rest of the house or objects in the house, multiple transmitters provide alternate paths for the signal to enter the house and allow reception.
There are advantages for outside antennas as well. In urban areas, high-rise buildings will create shadows where the direct path to a single transmitter is obscured. If there is sufficient signal reflected from other buildings, it may be possible to use the multipath and a sophisticated state-of-the-art receiver to acquire the signal. However, if the signal is too weak, reception won't be possible.
In mountainous areas, it may be difficult to find one location capable of serving all the population centers in the area, since they are often located in valleys. Multiple transmitters can fill in the gaps.
CHALLENGES TORECEPTION OF MULTIPLE TRANSMITTERS
Why hasn't this technology been adopted in the U.S.? If you followed the 8-VSB versus COFDM debate a few years ago you already know one of the reasons. COFDM can be easily configured to handle the multiple time delayed (multipath) signals present in a SFN. Poor performance in the presence of multipath has been considered one of the main weaknesses of the 8-VSB modulation used in the ATSC system. That is changing. Advanced technology like Casper, used in the Linx 8-VSB receiver, does a much better job handling multipath. Given the superior carrier-to-noise performance of 8-VSB ATSC modulation, with this technology, 8-VSB should be able to perform better than COFDM in many situations, even in the presence of multipath.
There are two ways an 8-VSB receiver can handle multipath. If the multipath, either from a reflection or another transmitter, is uncorrealated, then it will be treated as noise and thus will have to be at least 15 dB below the primary signal for even a chance to be demodulated. In the real world, a ratio of 20 to 25 dB is more realistic. On the other hand, if the multipath is within the delay range of the adaptive equalizer in the demodulator, it can be equalized. Advanced equalizers can even combine the energy from multiple echoes where carrier-to-noise (C/N) ratios are less than threshold to output a signal with a C/N above threshold. For maximum coverage, it is important that signals from multiple transmitters in the network arrive at the DTV viewer's antenna with delays and/or amplitudes within the correction range of the equalizer in the DTV tuner.
In the case of boosters, keeping the delay in the booster to a minimum and locating it in the direction as the main transmitter can reduce the problem, if the geometry of the viewer and the two transmitters allows it. If multiple higher-power transmitters are used to cover a more diverse population, this won't work unless timing is taken into consideration.
(click thumbnail)Map 1
(click thumbnail)Map 2
(click thumbnail)Map 3
SYNCHRONIZINGTRANSMITTERS
As noted earlier, if all transmitters in a SFN are fed from a common point, the timing at each location can be adjusted to minimize the "multipath" problem where there is an overlap in the coverage from two or more transmitters.
Merrill Weiss has been working on a practical SFN implementation for WPSX-DT in Clearfield, Penn. Part of it may be operational by the time you read this. Merrill was kind enough to send me some maps showing how SFN technology is predicted to solve reception problems for one station.
Map 1 shows the terrain-sensitive coverage with a single transmitter. The colors depict the predicted received signal level (RSL). Yellow is more than 80 dBu, which should be sufficient for indoor set-top reception in most cases. Orange is 70 to 80 dBu, which may be sufficient for indoor antenna reception if a tuner with a sufficiently robust equalizer is used. Red is used for the range between 60 and 70 dBu, usually enough for reliable reception with an outdoor antenna. Green represents an RSL between 50 and 60 dBu. This is getting close to threshold and reception will not be as reliable, if it is possible at all. Blue represents the signal level between 39.8 dBu (the noise-limited reception level after the dipole factor for Channel 15 is taken into account) and 50 dBu. Reception at this signal level will be even less reliable. Notice that while Clearfield should get a good signal, two large communitiesState College to the east, Johnstown to the south and Altoona between the two- are not expected to get reliable reception from the single transmitter.
In Map 2, the received signal level from the single transmitter and three distributed transmitters is shown. You will notice that all three communities that had trouble before are now predicted to have reliable reception.
What about the interference (multipath) created by the transmitters? Map 3 map shows the calculated interference between the transmitters. Only the yellow area has a carrier to interference (C/I) ratio greater than 20 dB. Outside this area, interference from nonsynchronized distributed transmitters could exceed the ability of the adaptive equalizer in the receiver to correct for it and make reception difficult, if not impossible. The interference ratio is worse for the orange, red, green and blue areas respectively. There are some areas between Johnstown and Clearfield, and State College and Clearfield, where it appears interference will be a problem.
By feeding the transmitters from a common source and controlling the timing, we can regain coverage in some of the areas where the C/I ratio is less than 20 dB. Although timing sounds simple, the geometry of the sites requires careful consideration. The transmitter locations, antenna patterns, power levels and timing have to be chosen so that the interference occurs away from populated areas.
A look at the ATSC signal coding standard reveals another problem, but I'll save that for next month when I'll tackle the complexities of single-frequency networks (both distributed transmitters and on-channel boosters) and report on some of the studies conducted to see how well it works outside the lab.
As always, comments, questions and suggestions are welcome.Drop me a note at dlung@transmitter.com.
The professional video industry's #1 source for news, trends and product and tech information. Sign up below.
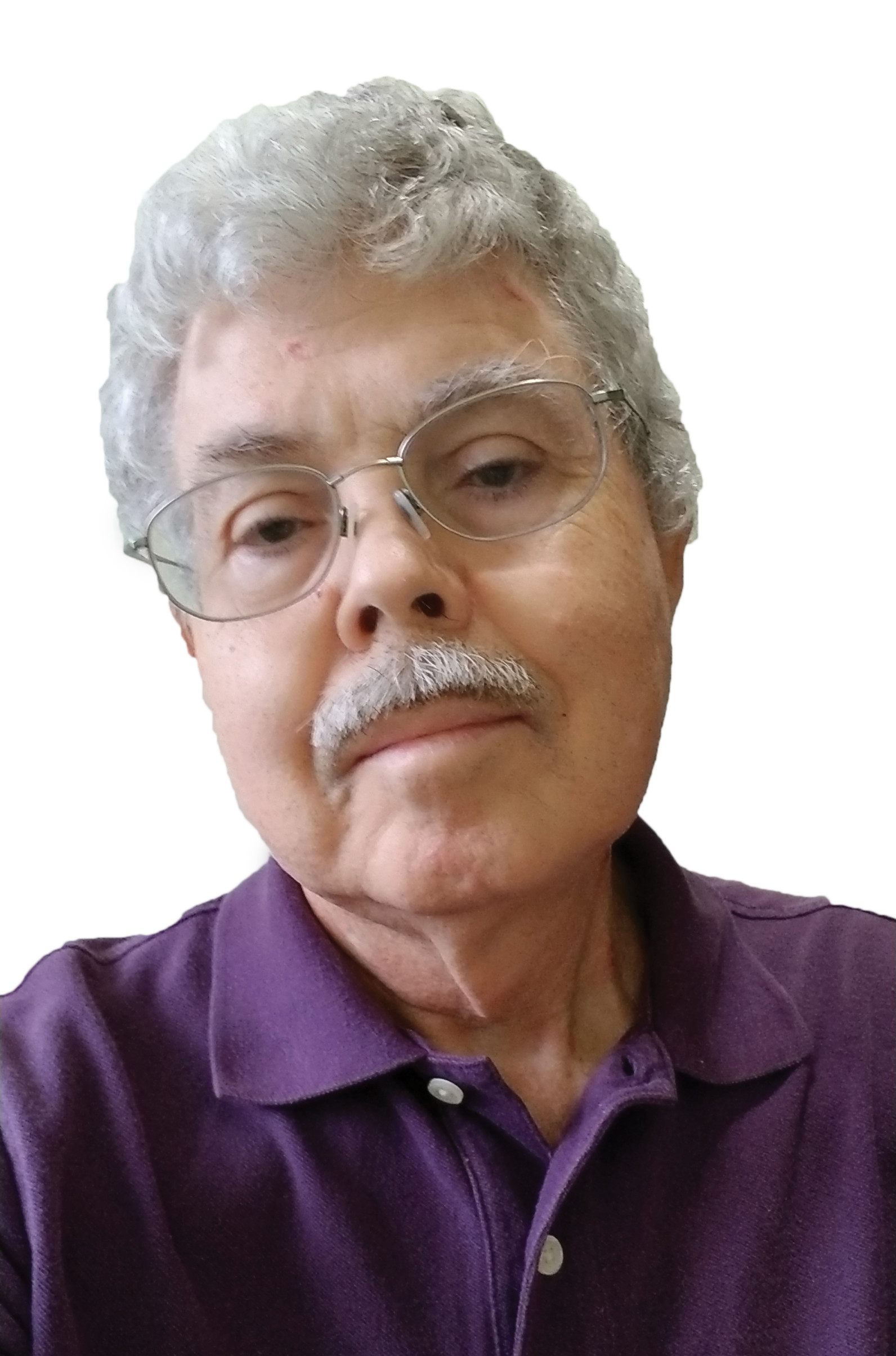
Doug Lung is one of America's foremost authorities on broadcast RF technology. As vice president of Broadcast Technology for NBCUniversal Local, H. Douglas Lung leads NBC and Telemundo-owned stations’ RF and transmission affairs, including microwave, radars, satellite uplinks, and FCC technical filings. Beginning his career in 1976 at KSCI in Los Angeles, Lung has nearly 50 years of experience in broadcast television engineering. Beginning in 1985, he led the engineering department for what was to become the Telemundo network and station group, assisting in the design, construction and installation of the company’s broadcast and cable facilities. Other projects include work on the launch of Hawaii’s first UHF TV station, the rollout and testing of the ATSC mobile-handheld standard, and software development related to the incentive auction TV spectrum repack. A longtime columnist for TV Technology, Doug is also a regular contributor to IEEE Broadcast Technology. He is the recipient of the 2023 NAB Television Engineering Award. He also received a Tech Leadership Award from TV Tech publisher Future plc in 2021 and is a member of the IEEE Broadcast Technology Society and the Society of Broadcast Engineers.