Methods of Information Delivery
The digital age is bringing to light a great deal more types of information and an equal number of variations in how to deliver it. Information, when considered in the context of the television broadcast perspective, is rapidly being categorized into as many forms as formats.
When information is communicated on a point-to-point basis, there is generally only one transmission point and one receive point. This is the case with devices such as cameras and videotape recorders, studio-to-transmitter IFLs (links) and other situations where there is one and only one path or connection.
Broadcast, sometimes referred to as point-to-multipoint, consists of one transmission source and multiple receive points. These conditions include a camera feeding a routing switcher, which feeds VTRs, production switcher inputs and the television transmitter itself. Broadcast transmission assumes no requirement for back-channel or backhaul, but certainly can and is being implemented for newer and expanded broadcast-type services.
DELIVERY REQUIREMENTS
Information sets, when referred to in form as "data," are routinely added to, superseded by, inserted into and even entirely discarded. In television broadcast, that delivery form is most often "real-time." Of the many forms, one attribute we must be aware of in considering delivery requirements is that real-time data will expire over some time frame.
As each successive set of new data is received, a memory buffer holds that data long enough to convey meaning. This buffer is both physical and psychophysical. It consists of the phosphor properties of the display added to the human eye and our mind's ability to perceive and retain that data. This information system, data as imaging information, continues to flow repetitively with only the image data content changing.
Real-time data, therefore, is characterized by the amount of data necessary to completely and accurately convey that message within some given time frame - after which its usefulness may be limited.
ALTERNATIVE APPLICATIONS
Alternative quasi-real-time applications are possible when the delivery method utilizes nonreal-time communications. These delivery periods need to account for additional characteristics or properties, some of which are nondeterministic. Nondeterministic time frames include the period needed for a communications channel to be established and are further influenced by the amount of bandwidth headroom available to deliver that information set. The Internet is an example of this quasi-real-time delivery application.
Faster-than-real-time (FTRT) delivery is also a subset of quasi-real time. We have heard much discussion about FTRT in the past several years, nearly all of which has centered on bit-rate-reduced video exchanges among video file servers.
Although possible, serial component analog video delivery could be thought of as FTRT because the two- or three-signal components (Y/C or YCrCb) making up the video signal could be synchronized at a faster rate and transmitted serially. Such concepts were used in MAC forms of satellite coding. Still, with the advent of digital technology, the hopes for greater-than-real-time analog video have all but vanished.
ITU-R 601
To realize where these information attributes come into perspective, consider a facility built around ITU-R 601 digital signal delivery and support equipment. Point-to-point intrafacility data delivery requires the full 270 Mbps of bandwidth on a single coaxial cable to enjoy the total value of the 4:2:2 signal at highest quality. However, if the signal can be losslessly compressed to 12 Mbps, it can be delivered in quasi-real time to a device that can, in near-real time, decompress and display that signal on a similar display device with comparable visual quality.
The additional time elements necessary to encode and decode the bit-rate-reduced signals are deterministic. If the signal must be delivered over the Internet or via a public network, connection time and other non-deterministic factors could render the signal less useful for time-sensitive applications.
Real-time data is generally considered to require a high-speed data connection. The data, in most cases, must be isochronous. In the case of video, interface requires a high-speed connection. In the case of real-time machine control, the data can be low speed in nature and still achieve its proper usefulness.
Received data must not be misinterpreted. Any information that is error-sensitive must not be compromised along the path between transmitter and receiver. The value applied to the sensitivity of errors is defined by the maximum acceptable symbol error rate permitted.
PULSE-CODE MODULATION
Error sensitivity can vary for certain parts of the same information. For example, in pulse-code modulation - the method used to transform analog information into a noise-free digital environment - the LSBs (least significant bits) are less error-sensitive than the MSBs (most significant bits) .
Error protection begins with the preparation of the data prior to sending it into the transmission path. In areas where only detection of an error occurrence is important, a small amount of data redundancy is added to the information in the form of a cyclic redundancy code (CRC). Used for decades, CRC became most recognized in computers where MS-DOS would add a checksum during the recording of data to the floppy disk.
File compression utilities (e.g., PKZIP) as well as modem protocols (e.g., XMODEM) utilize this method to signify, but not necessarily correct, data transmission-related errors. These concepts should not be confused when applied to video server RAID technologies, which use parity as a means of error recovery in the event of a failed disk drive.
There are some applications where only error detection is necessary. Such is the case when a reverse channel or back-control is available that will notify the transmitter source that received data was incorrect, sometimes referred to in computer language as "non-acknowledge" (NACK). The same data would then be retransmitted and the bad data replaced.
DETERMINING FACTORS
In the case of real-time applications, some of the determining factors for error handling depend upon the importance of the data, its uniqueness, whether that data will expire prior to retransmission or if the time available for correction is insufficient. Some data applications, particularly video and audio implementations, depend greatly upon the degree of error concealment that the receiver/decoder can account for and correct.
Videotape recorders rely heavily upon concealment (in conjunction with irregular interleaving or shuffling) as their correction method. The correction is achieved by the replacement of certain color-different pixels with adjacent pixels. Other recording methods depend upon the image or sound recovery and correction capabilities of the actual compression scheme.
Where the transmission or storage of information is unique or important, error correction is required. The means of providing that corrective data is dependent upon on the particular transmission or storage scheme employed. If the information is less important or will be superseded in a short time period by successive data (as is the case in emission signals for digital broadcasting), a different scheme may be employed.
In hostile environments where transmission errors are likely, where there is no means to signal back for retransmit, or the time to replace bad data is too high, an additional method of error handling is required. Forward error correction (FEC) is a method whereby during the source coding process, data is randomized and interleaved, prior to the application of transmission coding algorithms.
This effectively spreads the data out over time and reduces the probability of errors being induced into large continuous areas of the stream or image. FEC has a strength metric that defines the number of errored words that may be detected and in turn corrected over a given period.
ROBUST SIGNAL
FEC also makes the signal more robust and, like the other forms of error handling, requires additional overhead. Along with this increased bandwidth overhead, a short-term decoder memory buffer is needed to compensate for the time needed to receive, process and replace the errored data.
This latency thereby alters the real-time nature of the transmission and will vary with the equipment and degree of bit-rate reduction. Different forms and strengths of FEC are applied, depending upon the type or usage of the signal and the potential hostility of the environment in which it will be placed.
The science and applications of error detection and handling, while complex in nature, are becoming everyday real parts of the transition to digital television broadcasting. Understanding what form of error is occurring and how to recognize it, is becoming a part of the broadcast engineering technician's toolkit of knowledge. The concepts discussed in this installment can be applied to many elements of the digital television facility - including video servers, facility links, signal subsystems and emission.
Get the TV Tech Newsletter
The professional video industry's #1 source for news, trends and product and tech information. Sign up below.
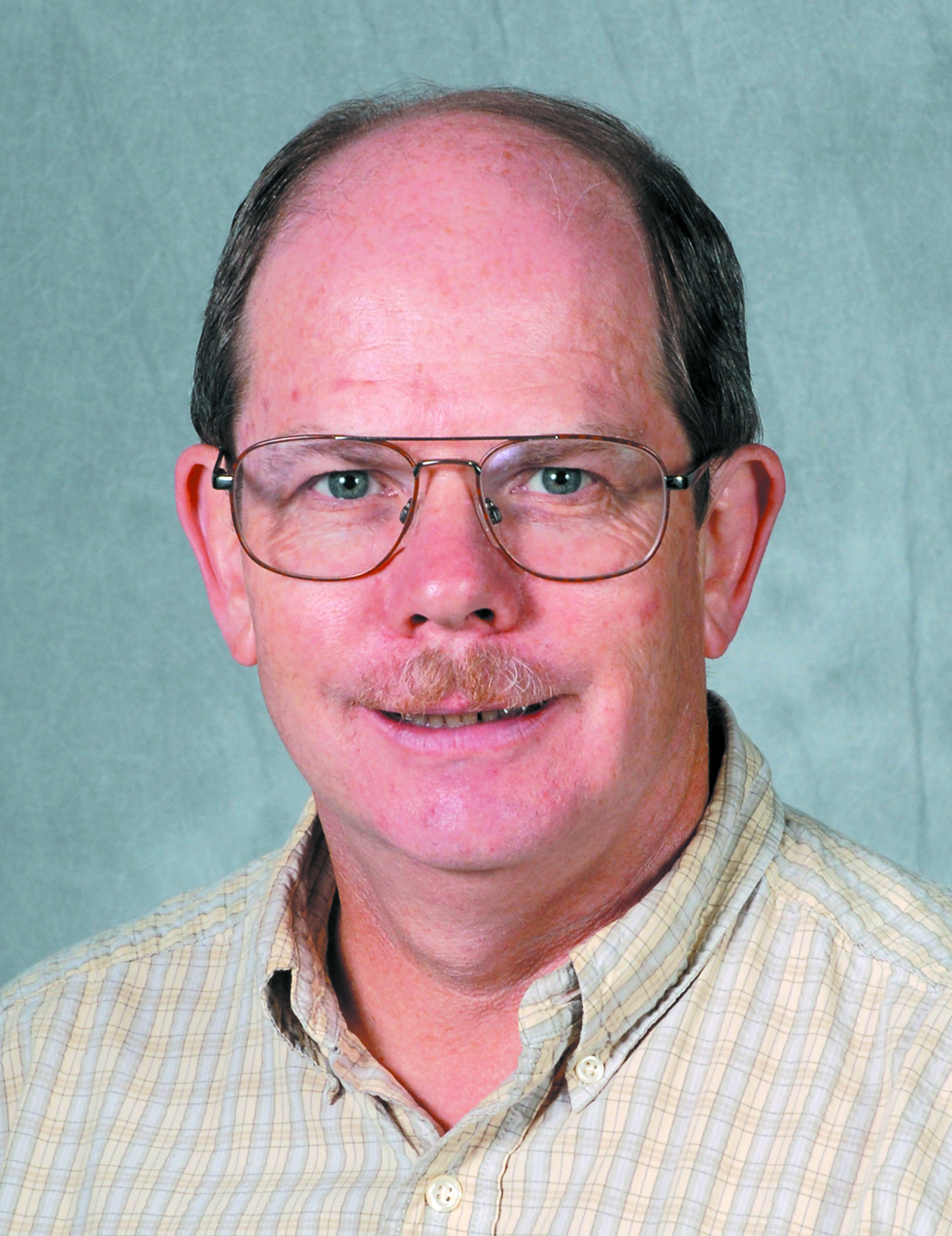
Karl Paulsen recently retired as a CTO and has regularly contributed to TV Tech on topics related to media, networking, workflow, cloud and systemization for the media and entertainment industry. He is a SMPTE Fellow with more than 50 years of engineering and managerial experience in commercial TV and radio broadcasting. For over 25 years he has written on featured topics in TV Tech magazine—penning the magazine’s “Storage and Media Technologies” and “Cloudspotter’s Journal” columns.