Achieving the New Technology Of Shingled Magnetic Recording
EIGHTY-FOUR, PENN.—The quest to achieve more magnetic storage in the same or smaller footprint continues as scientists and manufacturers strive to reach maximum technology potentials. And just as we thought, the physical properties of magnetic spinning disk drives were at their limits; hard drive capacities are doubling or tripling with chassis format sizes remaining essentially the same.
As of this decade, 3.5-inch drives have shrunk their read/write heads to about as small as can physically be built. Tracks are placed closer together and rotational RPMs have peaked to bearing and motor drive limits. To add more storage capacity requires that areal density—the bits per unit space— be further increased or that additional platters per drive assembly be developed; the latter resulting in huge costs and/or increases in the physical chassis dimensions.
If we believe that technology has reached its physical limits, then architectural changes to push capacities higher into the future may be the next order of business. Recent technologies have succeeded in areal density improvements through the use of Perpendicular Magnetic Recording (PMR), a parallel track layout with narrower bands (~75 nanometers in width) and a perpendicular alignment of the magnetic “bits” on the tracks. An engineering hurdle for PMR is it approaches crosstalk-thresholds, which result in “bit-flipping” or “flipped magnetization,” i.e., the magnetic bits effectively change polarity, resulting in data-errors (aka “bit-rot”).
5 TB AND BEYOND WITH SMR
In 2014 we saw “at or above 5 TB HDD capacities” (per Seagate) employing a technology, which changes the landscape of how magnetic tracks are recorded on HDD platters. The relatively new technology behind this evolution is called “shingled magnetic recording,” (SMR). Named for its appearance, tracks now resemble the overlapping of shingles on a roof. This process leverages other advancements in drive development, but goes even further; resulting in an 8 TB HDD (Seagate, March 2015), but intended for uses that differ from conventional HDD applications.
SMR drive applications are intended for archive purposes and fundamentally work as follows.
Fig. 1: Traditional disk drive track layout and recording process Historically, track width was defined by the size of the write heads. Head dimensions are established so as to apply just enough “bit-polarization” to the magnetic track such that read heads, which are typically smaller than write heads, can have sufficient magnetic pickup to recover the bits’ polarity alignment on the tracks, without interference, cross-talk, or misinterpretation from the adjacent tracks. As shown in Fig. 1, conventional recording polarizes magnetic particles on each track during the write-process. Track space, called “guard band” or “guard space,” insulates the data from each adjacent track. During read cycles, a smaller head is aligned to the center of the track; but, as diagrammed, this leaves “leftover” track space to compensate for drift, wobble and data-error-mitigation/recovery issues.
Fig. 2: Shingled magnetic recording (SMR) with its narrower and overlapping “trimmed tracks” SMR does two things: first, it shrinks the guard band; and second, it allows the next written track to overlap the previous track (see Fig. 2) like shingles on a roof. What remains is a “trimmed track,” containing sufficient data-density (magnetic strength) in a much narrower area, at a width sufficient for the smaller read head to recover the data completely.
PAYBACK AND COMPROMISE
Technological advances almost always have consequences. There are fantastic pluses in terms of increasing data-density when using SMR technologies; but the payback is in how the drive functions compared to traditional HDDs. Initially, when the SMR drive platter is “empty,” data is sequentially written onto the drive in tracks. Each next successive write overlays the previous (adjacent) track, but not enough to destroy the integrity of the earlier written data on the previous track.
Here’s the compromise. If the drive needs to re-write data over an already written track, its footprint will be wider than the trimmed track. The result is the next write obliterates the previous track adjacent to it. This only occurs when the drive writes to a series of tracks that contains previously written “legacy data.” Because the next write path is wider than the trimmed track, this new track destroys the data written on the adjacent track, rendering it useless.
On the surface, it appears that SMR techniques essentially give the drive a WORMlike (write once, read many) architecture. While data can be written in much higher density than conventional drives, and read back many times, it suffers when having to write new data over existing tracks, thus rewrites require a different approach. A technique employed by Seagate changes this perspective, with groups of tracks now written into bands. The end of each band breaks the shingled track layout, which modifies the track and allows a methodology to rewrite certain areas of the data track based upon control algorithms. This compromise means, in some cases, the overwriting of some sectors will require an entire band to be rewritten; however, this approach alleviates the requirement to rewrite an entire platter.
PERFORMANCE ADJUSTMENTS
SMR drives are designed for deep storage applications—whereby data is written but would seldom, if ever, be written over—such as an instruction to purge all the data on a platter. Since the rewrite process changes the performance of the SMR drive, it places the use of this drive into a category not unlike one would expect in a tape archive.
Take for example a photographic archive with millions of photographs that require rapid access at any time (e.g., a surveillance library, health-record images, and especially Facebook). Essentially this data is never modified; once written, it is intended only to be “read” at some later time. The value in using magnetic HDD (versus tape) is in its near instantaneous retrieval of data; mitigating the need for data migration or replacement of the physical storage media due to age.
Systems can further leverage drive spin down or “power-down” technology, where drives sleep until called into action, generally when a specific data track needs to be accessed, thus saving energy and prolonging drive life expectancy.
By further employing erasure-coding, as utilized in object-storage technologies, data can be spread across several sets of drives, arrays or in geographic locations—ensuring protection and resiliency should a drive fail to spin up again after sitting idle for long lengths of time.
The intelligence behind incorporating SMR technology into archive applications is fairly straightforward up to the point where tracks would be rewritten. Here a means to cache existing data on the soon-to-be overwritten tracks adds both secondary storage components and latency to the system. Manufacturers of digital archives are cognizant of these constraints. Users must be aware of their applications for SMR-based storage and tailor them accordingly, noting that SMR drives are specifically labeled for this application.
DEEP DRIVE ARCHIVES
SMR-drive technology is ideal for deep archives, which are seldom modified, such as in massive data centers or cloud storage such as Amazon Glacier. The longevity of these systems can be extended because drives won’t need to stay awake at all times. Furthermore, any migration path, which is necessary in aging tape libraries or as the technology for that era of tape drive elapses, is all but eliminated. Thus, SMR-archives become Tier 3 relevant as Tier 2 nearline storage-life-cycle management is maintained.
From the automation perspective, hierarchical migration based upon data-age or applications, transfers of Tier 2 data to permanent “deep-archive” occurs when the rewrite requirements are reduced to near-zero. Think again of Facebook whereby images are retained essentially “forever;” they are never written over, yet they still must be accessed relatively quickly based upon Friends who want to repost older pictures or videos months to years later.
Upcoming alternatives for archives remain. Gaining in popularity are opticaldiscs, which (today) still have much less data density than magnetic disks, but have fairly rapid access and tout data media life-expectancy in decades without data risk due to mechanical (i.e., HDD) failures.
Most recently, archive system manufacturer SpectraLogic introduced ArticBlue, employing SMR-drive technologies in a high-density, on-premises deep storage disk system. Cloud service providers, as well as OEMs, are surely expected to follow SMR technology well in the future.
Author’s note: Recognition is given to Seagate and SpectraLogic for their technological contributions to this article.
Karl Paulsen, CPBE and SMPTE Fellow, is the CTO at Diversified Systems. Read more about this and other storage topics in his book “Moving Media Storage Technologies.” Contact Karl atkpaulsen@divsystems.com.
Get the TV Tech Newsletter
The professional video industry's #1 source for news, trends and product and tech information. Sign up below.
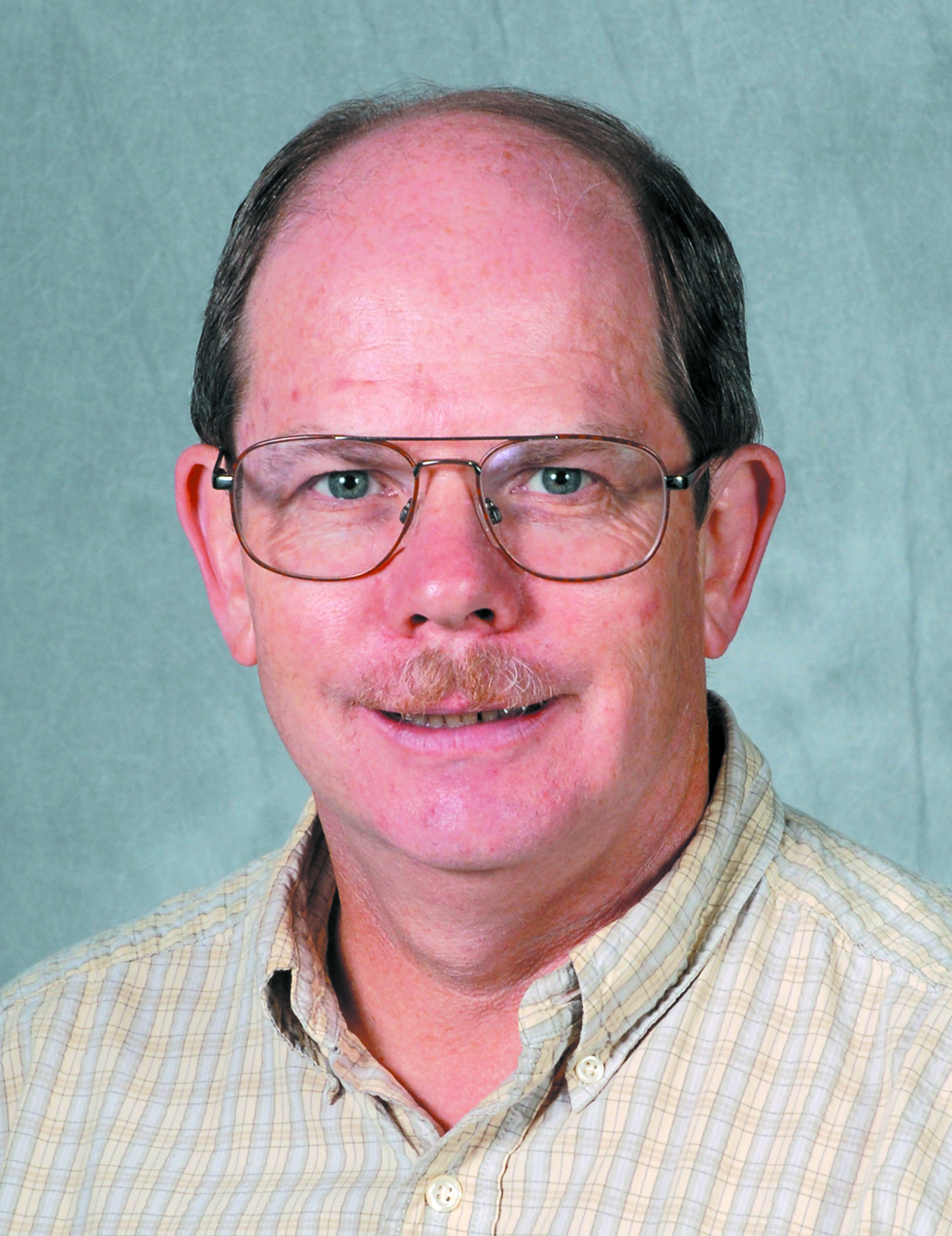
Karl Paulsen recently retired as a CTO and has regularly contributed to TV Tech on topics related to media, networking, workflow, cloud and systemization for the media and entertainment industry. He is a SMPTE Fellow with more than 50 years of engineering and managerial experience in commercial TV and radio broadcasting. For over 25 years he has written on featured topics in TV Tech magazine—penning the magazine’s “Storage and Media Technologies” and “Cloudspotter’s Journal” columns.